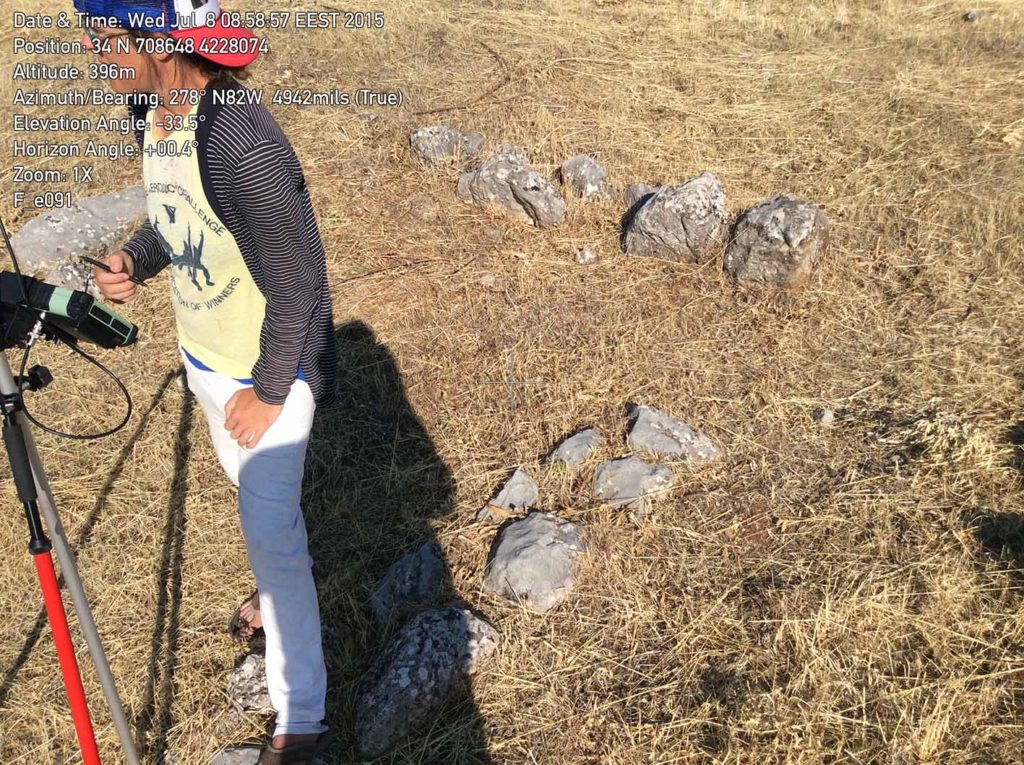
Gathering spatial data is a core aspect of archaeological fieldwork. Whether on a survey or an excavation, we spend a lot of time finding walls, artifacts, and other features, and the location of those things needs to be mapped, documented, and eventually published in the form of plans, elevations, maps, etc. Some measurement are best taken using tried and true analog methods, but if you want to acquire a lot of accurate spatial data or measurements, it helps to turn to technological solutions.
The thing that I’ve been in charge of most often on survey and excavation projects is using these sorts of technological solutions to acquire and manage spatial data. I don’t feel like I’m all that old, but it is pretty amazing how much this technology has evolved since I started out on my first project in 2003, and even in just the last five years. BEARS team members will know that we’ve been using a new EMLID REACH RS+ dGPS unit to acquire spatial data on our project and that I am very enthused about this product and its implications for archaeological fieldwork. To put that excitement into context for everyone, I thought it would be helpful to review the kinds of options for gathering spatial data I’ve used in my career, and why the EMLID is such a game changer.
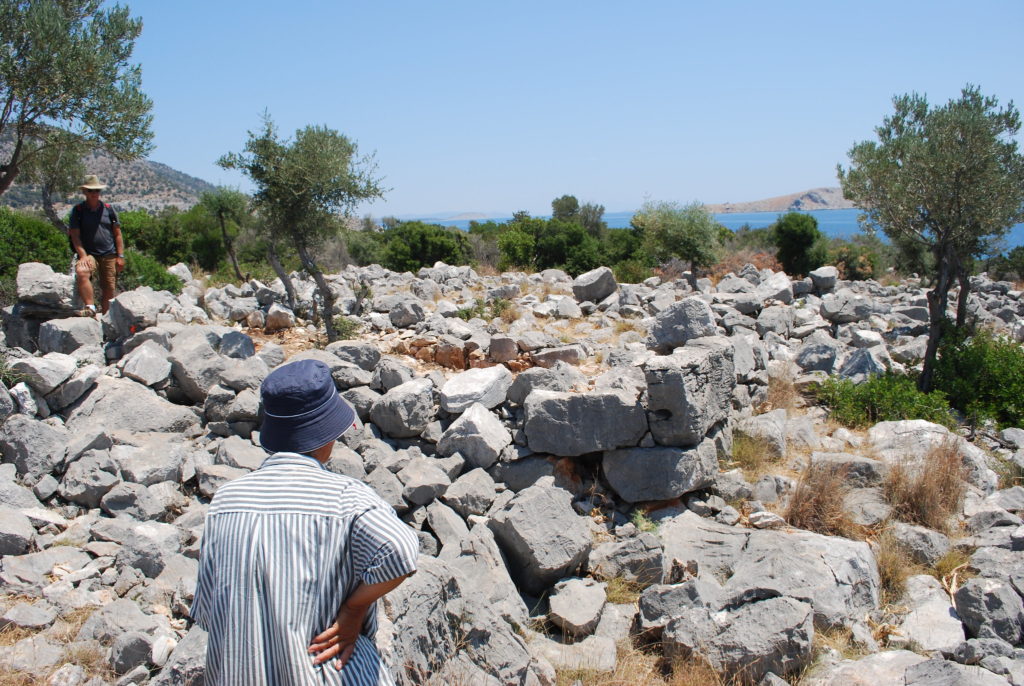
If you have worked on an excavation project, you will probably be familiar with what is still the best tool for gathering accurate spatial data rapidly – the Total Station, aka Theodolite, aka EDM (Electronic Distance Measurement) unit. A Total Station is an instrument that uses optics to measure distance, equipped with an internal computer that triangulates positional data. Once a Total Station is set up, it is easy to take hundreds and hundreds of readings from it very rapidly. The data can be very accurate.
However, Total Stations are basically useless for survey projects – the setup requires triangulation from highly precise reference datums, and you are obviously not going to have a dense network of precise datums throughout a whole survey region. The setup for a Total Station is time-consuming, so even if by some miracle you had good datums everywhere, you’d be wasting huge amounts of time setting up the station every time you found a random wall or foundation in a unit. Maybe most importantly, the Total Station is heavy and bulky, so not a good companion for field traipsers.
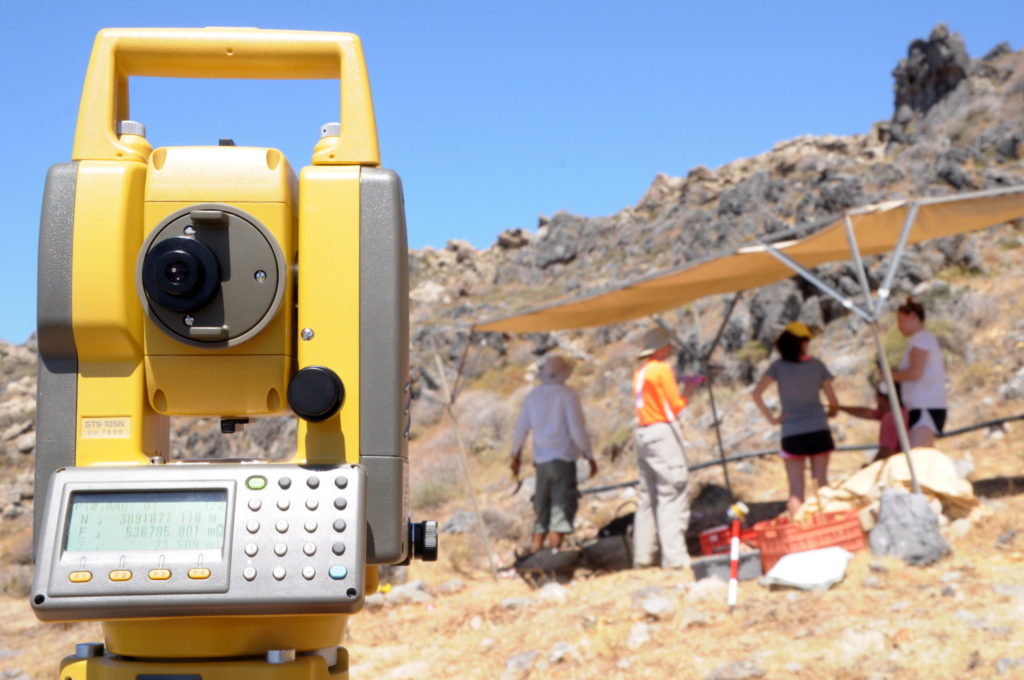
Archaeological projects at the level of the region usually employ GPS technology instead. GPS provides location data by calculating distance from a receiver to at least four known satellites’ positions based on the time required to transmit a signal between the receiver and the satellites. It then triangulates the receiver’s position based on those distances.
On my first survey project, the SHARP project around Korfos in the eastern Peloponnese, handheld GPS units were being used to map architectural features. Handheld GPS units were – at that time, ca. 2008 – cheap and widely available, so at first glance quite well suited to survey. However, handheld GPS units are not great when it comes to data quality. Like most basic GPS units, they use a kind of technology called Single-point Positioning (SPP). It’s just what it sounds like – the GPS position you get on the readout is based on the calculation of a single point, a single calculation of the GPS receiver’s position based on data from whichever satellites that GPS receiver can receive data from.
SPP is the most common form of GPS measurement (this is what the location data in your phone, your car, or hiking GPS is based on), but also the least accurate. A single-frequency GPS calculation of position is subject to a wide variety of errors, especially ones arising due to atmospheric distortions that impact the reading of distance from the receiver to the satellites. As a result, SPP GPS data is not very precise – usually you will know where you are within a range of 3–5 meters.
This is obviously fine if you are trying to find the nearest smoothie joint or stay on a hiking trail, but not so great for archaeological fieldwork. We learned this the hard way at SHARP – there were a lot of walls in the survey area, but most were not much longer than, say, 3–5 meters. Given the large error range of the GPS units, the “maps” we made of walls and buildings by taking points with handheld receivers looked more like children’s scribbles than like scientific documentation. And walls were close together, so it was usually impossible to tell, from the GPS-generated GIS map, which wall was supposed to be which when we went back in the field to check and refine the data. It was a real nightmare, and we ended up having to go back and basically start everything over with a new system, including renumbering most of the walls.
This is not to say that SPP GPS is useless for survey archaeologists. The receivers are small, easy to use, and fit in your pocket – I still use them all the time to record the general location of a feature in the landscape, remember the best path through maquis patches, or just get a handle of where I am while wandering around. But SPP GPS is demonstrably inadequate as a solution to the problem of precise (2-4 cm accuracy) geospatial recording in the context of archaeological field projects.
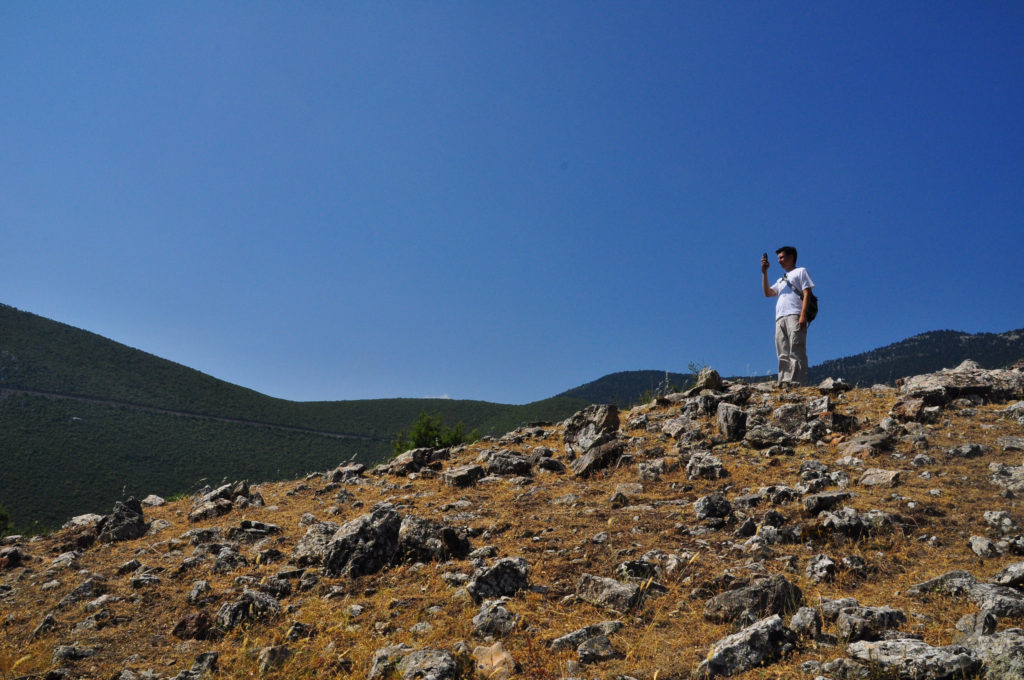
For sub-meter measurements, differential positioning is necessary. Differential GPS requires that two separate GPS receivers be set up nearby one another, tracking the same satellites. One receiver (the base station) is installed on a known point (a datum), and generates differential correction data according to calculations based on disparities between its known location and the raw satellite readings of its location. Another receiver (the rover) is used to collect positional data, which is then refined based on the corrections calculated by the local base station to reduce error. When these refined readings are being received by the rover in real time, so that the coordinates you are seeing on the rover interface reflect the corrected rather than the raw data, that’s called real-time kinematic (RTK) dGPS.
At SHARP, the project decided that the best solution to its substantial mapping needs was to bring out an RTK dGPS system to document all of the project’s architecture. We basically scrapped a lot of the work we had done with the handheld GPS units. Remapping everything with the dGPS was quite laborious. It is more time consuming to map with dGPS than with a Total Station, because with dGPS you want to take a bunch of readings and average them together, which irons out any randomly bad satellite data that might come down the line for various reasons. To get 2-4 centimeter accuracy data you should let the unit chug on a point for a few minutes. But we finally did end up with precise and accurate maps of quite a lot of architecture.
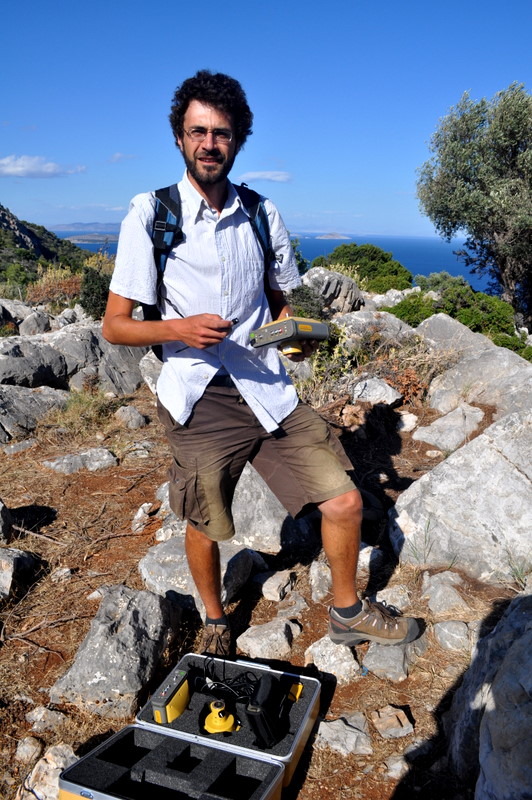
While a base + rover RTK dGPS system was crucial to the success of the SHARP project, it is not totally ideal for regional survey projects for a number of reasons.
First, the corrections data provided by the local base station decreases in value as a function of the distance of the rover from the station. Since errors from atmospheric distortion vary within relatively small areas, the relevance of the corrections data calculated by the base station to the raw data collected by the rover will decrease if the rover is collecting data outside of the immediate area of the base station. Accuracy is likewise impacted by the topography, especially if communication between the two stations is hindered by vegetation or terrain.
Unless project personnel can be spared to guard the base station while dGPS survey is underway, roving for data collection beyond the immediate, intervisible range of the local base station will entail a certain tolerance for the risk that this expensive equipment will be damaged by passersby (sheep and goat herds are common in Greek survey areas), weather events (e.g., strong winds that could topple the station), or mischievous puppies, etc., or even stolen while personnel are away from the station. Absent adequate tolerance for this risk, working with a dGPS system dependent upon a local base and rover setup will require frequent setup and teardown of the system, a time-consuming process that is also sufficiently complex to require expert operation and therefore additional training needs for project staff.
These systems have traditionally been relatively expensive (usually in the $20,000 range) because of the need to purchase two separate high-grade GPS receivers. If you are a small survey project without a ton of architecture to map, it’s not easy to swallow laying out that much money for accurate mapping.
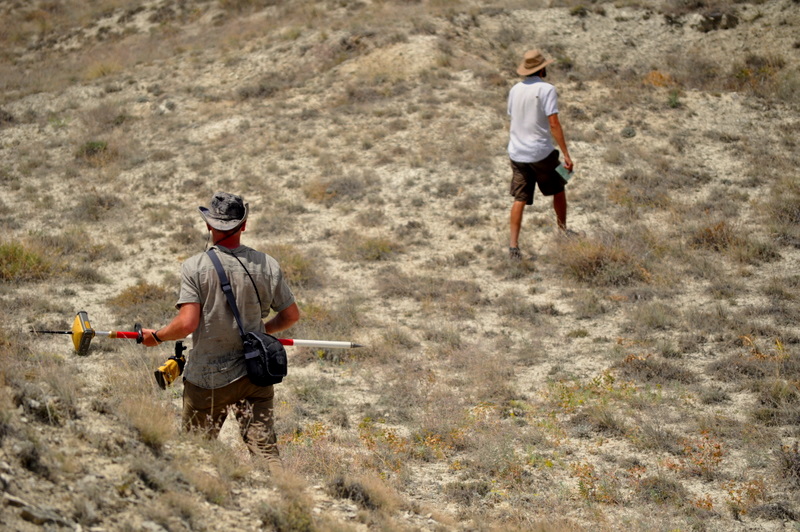
When I joined up with the Mazi Archaeological Project (MAP) in 2014, I was asked to take charge of the project’s mapping and photogrammetry needs. This was several years after the end of the SHARP project (I had last worked there in 2011) so I thought I’d look into what kinds of mapping options existed beyond the base + rover dGPS system we’d used at SHARP.
In doing so, I learned that the industry standard for dGPS had turned to a new system called WADGPS. WADGPS replaces the local base station with a distributed network of reference stations broadcasting regionally refined corrections data (they are based on area-wide differential calculations) to receiver stations connected to the network via wifi. In other words, with WADGPS the base station is replaced by a centralized network, so the user only needs a rover. WADGPS has existed since the late-1990s but had only recently become standard because of the proliferation of reference station networks. In Greece there are now several.
WADGPS obviously has a lot of advantages for survey projects. Since the generation of corrections data in a WADGPS system is centralized, the equipment required for survey is limited to a rover station, eliminating roughly half the cost of an initial investment in a dGPS system. The lack of a base station furthermore eliminates any concerns of mobility during the survey.
The WADGPS system also requires considerably less expertise to setup and operate than a base and rover system. Once the unit is configured correctly, the user may merely turn the unit on, open the survey software, connect to the RTK correction network, and begin high-precision survey in less than a minute.
Most importantly, experimental studies of the comparative accuracy of the two demonstrate that the distributed corrections data is ultimately more reliable in terms of precision over a wide range of spatial extent than a local base station’s corrections data.
I thought all this sounded really promising, so I decided to use some of my research funds to purchase a WADGPS setup for the MAP project. I settled on a system built around a Leica unit called the CS25. In principle, the CS25 setup seemed awesome – it was extremely lightweight and easy to transport. The handheld CS25 computer weighed very little. Besides that, all I needed for accurate survey was an aluminum pole fitted with a GNSS antenna and a SIM card with wifi that connected to the Leica SmartNet signal. When I picked the gear up and tested it out, I was really psyched about its potential. It seemed totally ideal for survey and mapping at remote sites like the ones we had at MAP, which had to be reached on foot through difficult and overgrown terrain.
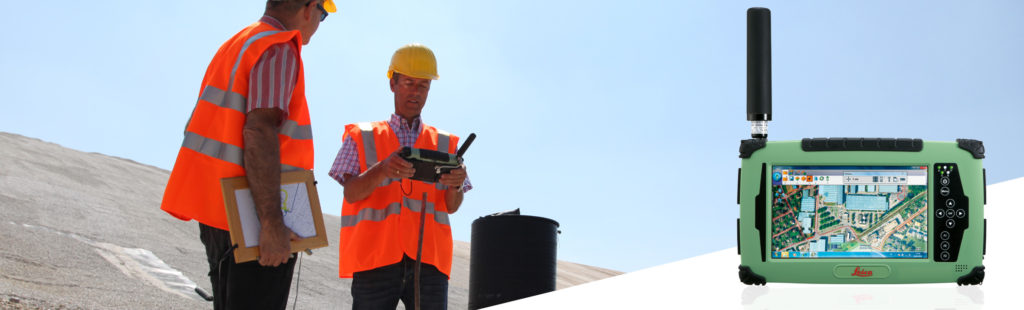
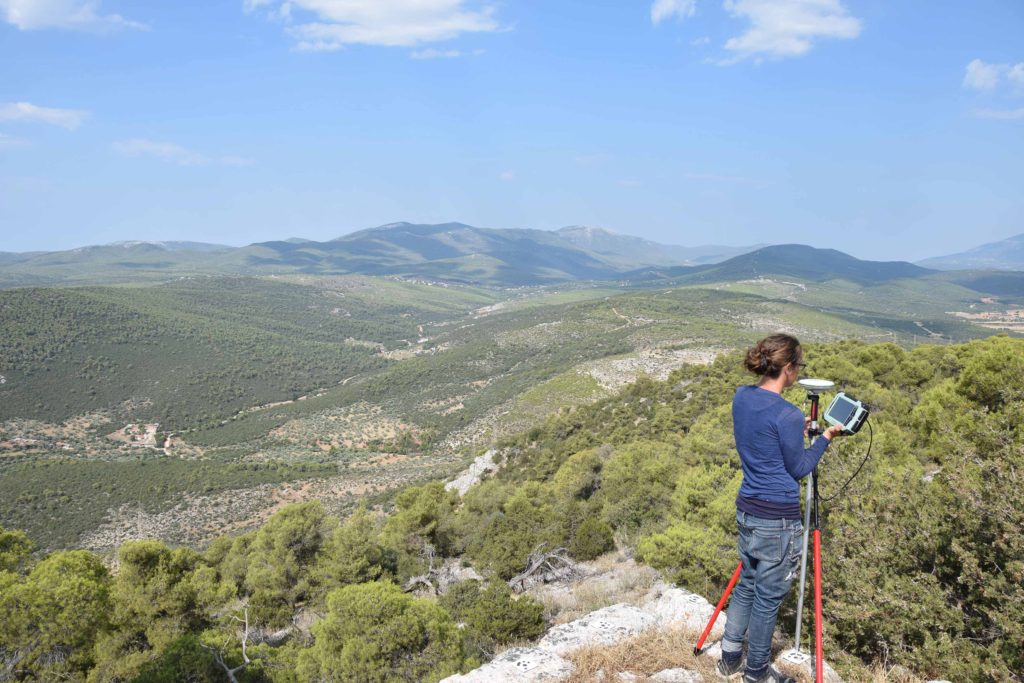
In practice, the honeymoon was over more or less as soon as I got the unit out into the field. I won’t go into the tedious details, but the CS25 the Leica representative in Iowa sold us (I was at Nebraska at the time) was physically a real piece of garbage, and the computer had all kinds of software bugs that made it go haywire. Often the unit would fail to connect to the GPS receiver at all. I spent a lot of time “turning it off and then back on again” and even more time driving to the Leica office in Athens to ask the techs there to update the software or figure out why we kept having connectivity problems. There is a set of uninsulated, high tension electrical wires that runs right over the site of Eleutherai where I was working a lot, and I developed a paranoia that those wires were somehow frying the CS25 motherboard or interfering with its mojo. So, yeah, the thing drove me kind of crazy. It was sufficiently bad that when I returned to Nebraska after the first summer of use in 2015, I sent it back to the Leica rep and told him he must have sold us a Lemon. And actually that was true! Dealing with that Leica rep was a total nightmare from start to finish – he did a lot of gaslighting to try to convince me that it was my stupidity rather than his crappy gear that was causing the malfunctions. But I eventually convinced him to send us a new CS25 to use in 2016.
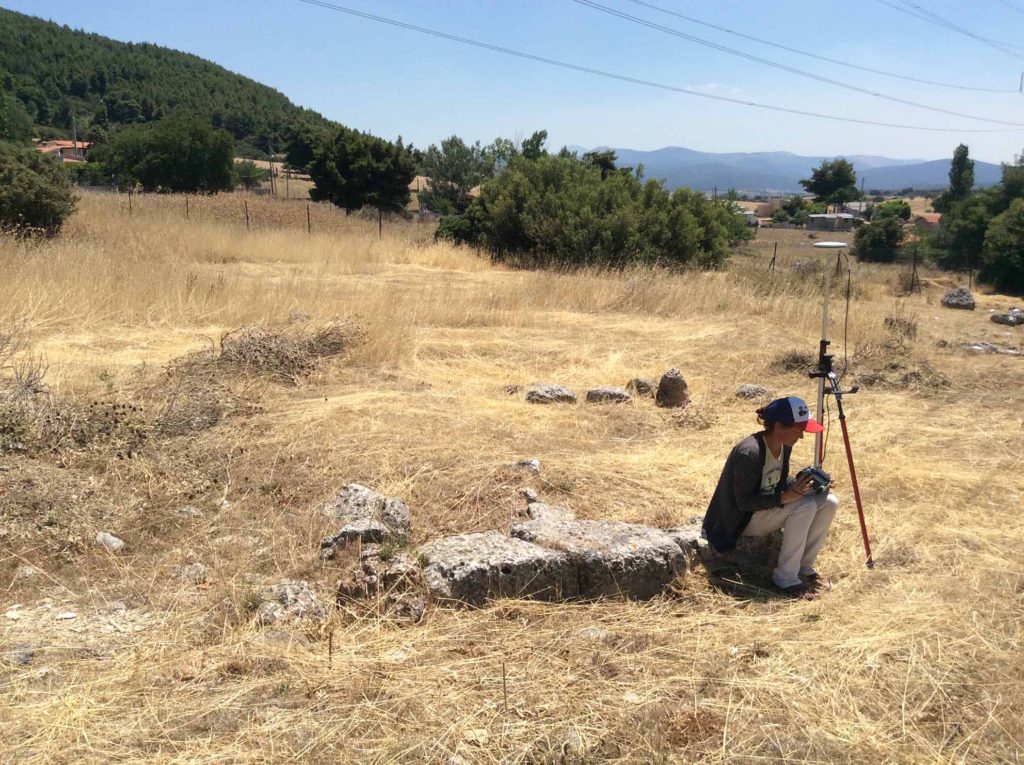
Fortunately that new unit worked much better, probably how it was intended to work from the beginning I suppose. When it was working correctly, it was as awesome as I had imagined. It was not a burden to carry around – I hauled it up many a mountain slope – and so easy to use. It really was just a matter of turning the unit on, opening up the software, connecting to the SmartNet, and BAM – before you know it you are collecting very accurate spatial data. We mapped a lot of architecture with the Leica in 2016 and 2017, and also used it for generating datum reference points for many photogrammetric models. My favorite part was mapping a bunch of rubble architecture that had been revealed by a recent forest fire, so working in an area of fresh burn. This involved weeks of fighting through bristly, charcoal encrusted maquis tentacles. I would return to camp everyday looking like a coal miner rather than an archaeologist.
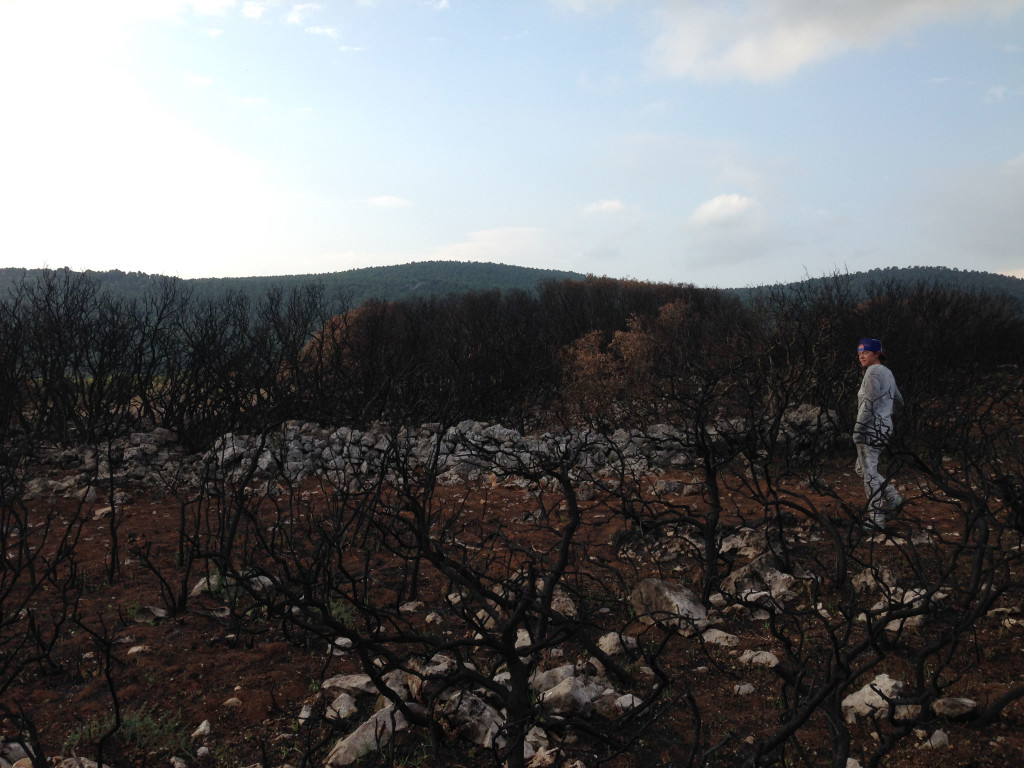
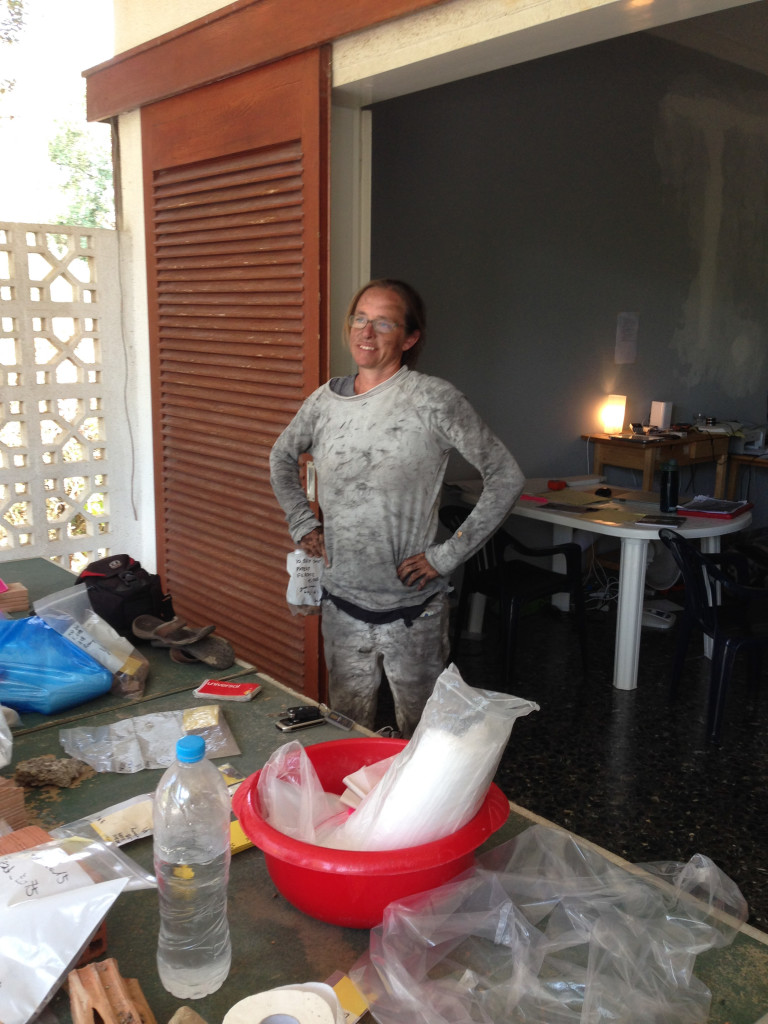
I guess you could say that my experience with the Leica unit was mixed. In the end it was a good solution and we did a lot of useful work with it at MAP. But it was still really expensive – over $11,000, plus the cost of subscribing to the Leica corrections data, a few hundred Euros a season.
But probably the worst part was actually the process of acquiring it in the first place: a hugely time consuming and confusing ordeal, that seemed much more like buying a used car or something than conducting a straightforward business transaction.
When I was putting together the purchase in 2014/2015, the only vendors were the big optics companies that make and sell Total Stations – Leica, Topcon, Nikon, Sokkia, etc. They are used to doing business with industrial clients that have nearly unlimited budgets and who often put in bulk orders worth hundreds of thousands or millions of dollars. They definitely do not really put a lot of care into customer service when it comes to small guys – like a single academic purchaser looking to buy one unit. Moreover, there is no actual price listed anywhere for the different units – you have to get in touch with a salesmen and ask for a quote.
Because the salesmen are like used car salesmen, they throw a bunch of extra bloat into the quote – adding in stuff like extra battery chargers and software packages that you will definitely never need – so you have to go back and forth with them to pare the inventory list down to what you actually want.
Also, because I was purchasing the unit through my university and it was over a certain dollar amount, I was required to get multiple competitive bids from different companies, so I went through the whole used-care salesmen process with several smarmy salesmen. Then, I had to play the different bidders off of each other to try to convince each one to bring the prices down. Anyway, it took forever and was extremely unpleasant. But there weren’t any other options – these companies basically had a monopoly on units of this kind, so there wasn’t much you could do but deal with it.
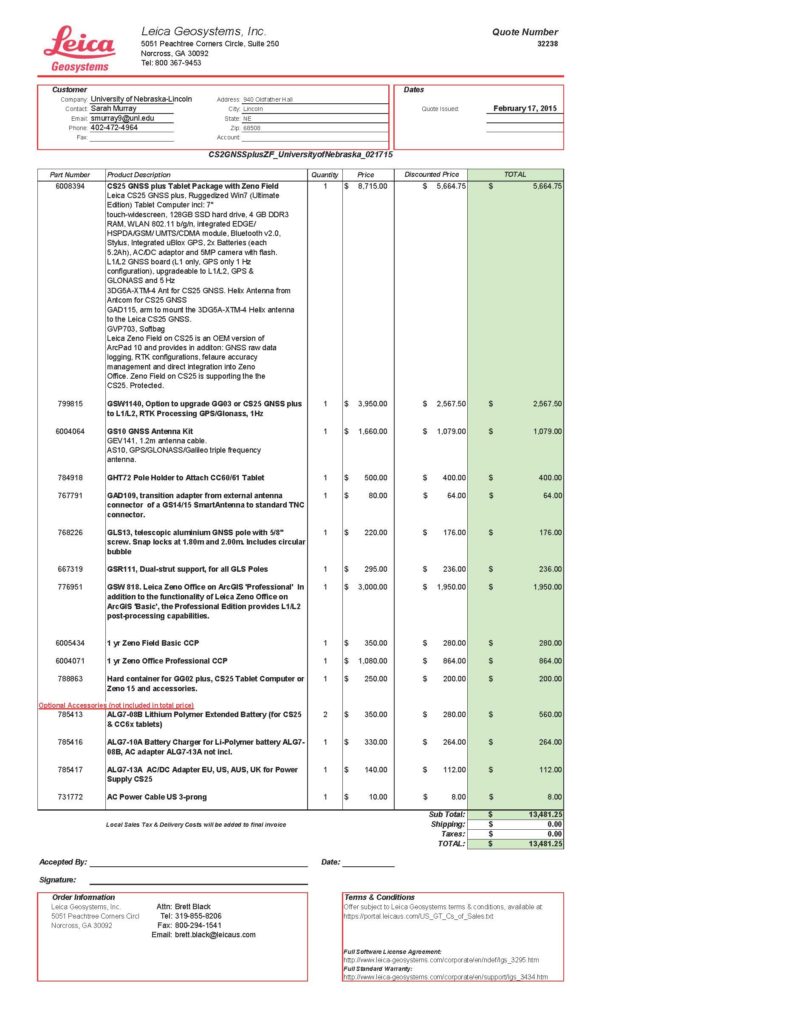
By the time I was starting the BEARS project, I had left my job at Nebraska, and the university-owned CS25 unit, behind. When I looked into purchasing a new unit for the new project, I found something really, really, really awesome. A small, independent startup company called EMLID had stepped in to “disrupt” the dGPS unit market – a totally corrupt and monopolistic corporate system where a bunch of behemoths controlled access to super accurate spatial data capture equipment.
Usually I hate anything to do with Silicon Valley corporate power-speak cliches like “disrupting”, but the EMLID units are nothing short of a revolution for small guys like archaeologists who just want a simple, elegant, inexpensive solution for capturing accurate GPS coordinates.
The EMLID REACH RS+ system does the same thing as the Leica CS25 setup, but better. Instead of using a propriety data processing computer, EMLID runs from an app that’s free to download on a tablet or smartphone, so you need to bring even less equipment into the field. In 2019 I did a lot of mapping from my iPhone, which is about 5% the size and weight of the CS25 unit.
It is cheap! Really cheap. The setup we got – which includes two high quality GPS receivers – was less than $2,000! And – wonder of wonders – the price is just listed right there on the website, no haggling or used car salesmen involved. The thing is so cheap that it doesn’t require any bidding through a university purchasing procedure. Really, it’s so cheap that you could just buy one of these out of pocket without losing a lot of sleep over it.
The EMLID system is also super flexible. Since we got two receivers, we are free to set the thing up either as a rover/base station system, or to use WADGPS if we decide we’re going to do enough mapping to merit subscribing to a distributed corrections data network.
Finally, the app is super intuitive to use and pretty much always worked as it was supposed to during the 2019 season. There are some small drawbacks – as of last year the unit didn’t allow a lot of flexibility in terms of the coordinate system in which you collect and display data – but those will probably be ironed out over time, provided the company is successful and commercially viable going forward.
If it is, I think it’s not going too far to say that we’ve entered a kind of golden age for accessible, high-quality spatial data acquisition in archaeology. I am pretty sure that the EMLID people did not intend to help us archaeologists out so much – it seems more like the market is people who make drone videos or something like that – but thanks to them I have hope that none of my junior colleagues will have to deal with a Leica used car salesman type of situation again.
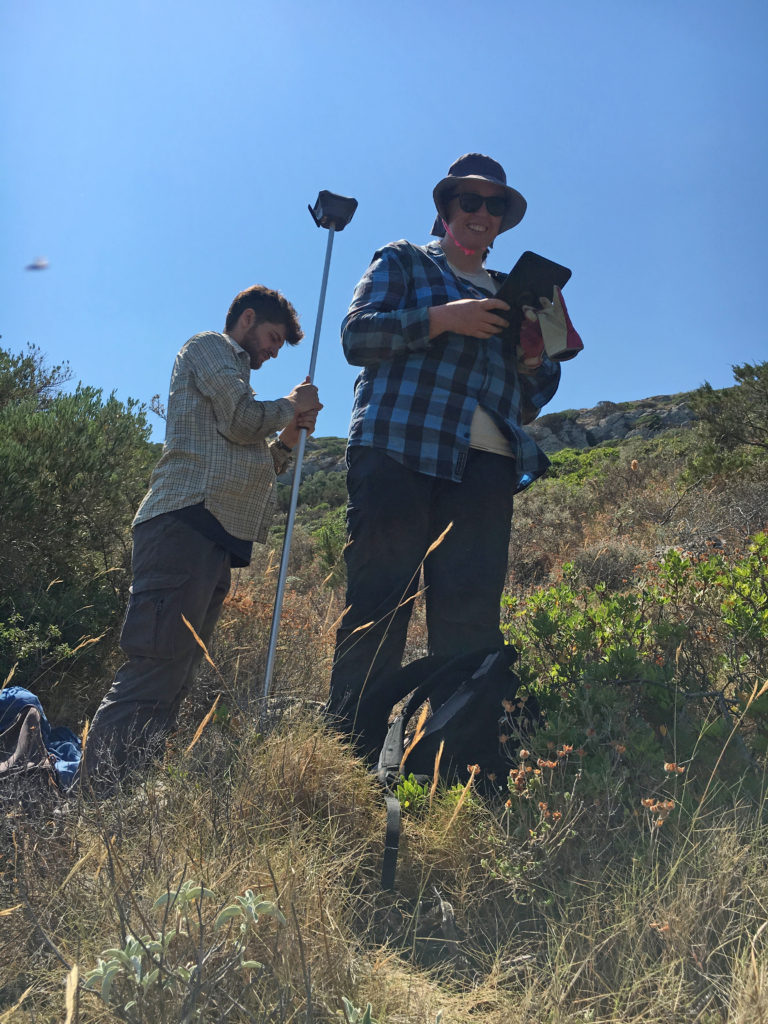